Why is Soil Biology Important? | Measuring Soil Biology | Management and Soil Biology | Soil Evaluation of Biological Productivity
There are two types of soil regulators – primary (or those associated with climate and geochemistry) and secondary (or anthropogenic regulators). Examples of primary regulators are soil water, temperature and soil type and these are highly interrelated. We as humans, have little direct control over these regulators. On the other hand, secondary regulators are directly influenced by human interactions with the soil – hence they are known as anthropogenic regulators. Examples of such regulators may include: organic matter quality and quantity (which might be determined by the crop type and sequence of cropping); soil disturbance (which would be directly affected by tillage) and inputs to the soil (for example: pesticides, fertilisers, animal manure and urine, lime and innoculants).
Primary regulators
Soil water
When the soil gets too dry, plant transpiration drops because the water is becoming increasingly bound to the soil particles by suction. Below a certain point, the wilting point, in agricultural settings, plants are no longer able to extract water. At this point they wilt and cease transpiring altogether. Such conditions are common in arid and semi-arid environments. However at this point (-1500 kPa) microbial processes still occur at reasonable level (about 35% of total activity). This reflects the adaptive nature of soil microbes as well as the fact water held in micropores is available for the soil microbes but not for plants. Importantly though optimum aerobic microbial activity occurs when the soil water potential is about -50 kPa or field capacity. Fungi are generally more tolerant of lower soil moisture than bacteria as bacteria tend to be less mobile, relying on diffusion to obtain nutrients.
Soil water has a large influence on the macrofauna inhabiting a soil habitat. Many reports suggest earthworm abundance to a 10 cm depth is influenced by annual average precipitation where there is a positive correlation between increasing annual rainfall and the number of earthworms in top 10 cm depth across a range agricultural field sites. This information is not surprising considering earthworms reliance on soil moisture to provide a moist external respiratory surface to facilitate gas exchange. Not to mention the effect drying would have on soil structure and the consequent ability of earthworms to maintain mobility in the soil environment.
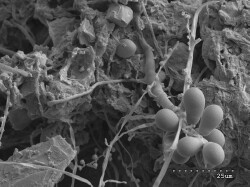
This figure shows fungal spores budding from hyphae. These structures can help the fungi survive adverse conditions due to drought, heat or chemical fluctuations. |
Temperature
Very few soils maintain uniform temperatures in their upper layers; variations are highly seasonal and diurnal however temperatures below the surface are far more moderated. Thus, diurnal fluctuations of 35°C at the surface are reduced to fluctuations of about 10°C at 10 cm depth and just 2°C at 20 cm depth. Factors affecting the rate of soil warming are the intensity and reflectance of solar radiation, degree of shading, surface cover, etc.). To highlight the interrelatedness of primary soil regulators - wet soils are far less subject to diurnal fluctuations than dry soils. Temperature affects a range of processes carried out by soil biota: for example - increasing temperature enhances mineralisation of soil organic matter by increasing reaction rates and accelerating diffusion of substances (nutrients).
|
In order to overcome or survive unfavourable conditions such as heat or dry, bacteria and fungi produce spores which generally have a protective outer coat. These spores are metabolically inactive and can survive for extended periods of time in such unfavourable conditions allowing the organism to “re-emerge” and continue normal growth cycles when conditions become more favourable. Up to 15% of the dry weight of a bacterial spore consists of calcium dipicolinate within the core, which is thought to stabilize the DNA. Dipicolinic acid could be responsible for the heat resistance of the spore, and calcium may aid in resistance to heat and oxidizing agents.
Soil type
Strong correlations exist between soil texture and the abundance and specific activities of the soil faunal and microbial communities. Soil microbial biomass and earthworm abundance generally increases with clay content. This is likely to reflect the increased water and organic matter content associated with soils containing more clay.
Secondary regulators
Organic matter
Aside from primary regulators, secondary regulators, directly influenced by human interactions, affect soil biology. Soil organic matter (SOM) quality and quantity (which might be determined by the crop type and sequence of cropping) is one such regulator. This may well be one of the most important soil regulators as SOM essentially provides the base energy source (carbon) for the entire soil biology ecosystem and as such the abundance and activity of soil microbial and faunal populations increases with increasing SOM. Multiple ecological studies have shown that above ground diversity influences below ground diversity and agroecosystems are one such example. Crop type and variety affect microbial composition and activity due to a number of factors: e.g. differences in the rhizosphere; differences near crop residue; direct effects due to chemical and biochemical properties (e.g isothiocyanates in canola) and indirectly through management.
C/N ratio
- C/N ratio influences mineralisation of N
- C/N ratio >25:1 stimulates immobilisation:
- C/N ratio <25:1 stimulates mineralisation
| Organic material | C:N ratio |
Soil micro-organisms | 8:1 |
Sewage sludge | 9:1 |
Soil organic matter | 10:1 |
Lucerne residues | 16:1 |
Manure | 20:1 |
Corn straw | 60:1 |
Grain straw | 80:1 |
Crude oil | 400:1 |
Pine wood | 625:1 |
The C:N ratio is a useful determinant of biological turnover of nutrients (e.g. nitrogen).
The C:N ratio of organic residues is extremely variable and at the same time important in terms of the energy budgets for the breakdown of various residues. The C:N ratio influences mineralisation of organic nitrogen in the soil. A C:N ratio >25:1 stimulates immobilisation of nitrogen while a C:N ratios <25:1 stimulates mineralisation of nitrogen.
Cultivation
Tillage and SOM availability are highly interrelated and have a significant effect on soil biota. Tillage affects SOM build up in two ways: i) through physical disturbance and mixing of soil which leads to exposure of soil aggregates to disruptive forces and alteration of physico-chemical properties such as aeration and water content. ii) through incorporation and distribution of plant residues in soil profile.
When we compare incorporated (tillage) residue to surface placed (no-till) residue a number of characteristics are generally observed for each management type. When residues are incorporated we observe: Faster decomposition and nutrient mineralisation (potential for leaching), more fast growing populations especially bacteria sp., fungal hyphal network damage, poor aggregation and soil structure, and less potential for SOM build up. While in surface placed residue (no-till) we see: slower decomposition and nutrient mineralisation, more slower growing populations, more fungal growth, better aggregation and soil structure, and better potential for SOM build up.
| |
This figure shows incorporated residue on the left with a decline in fungal hyphae compared to surface placed residues (right) which have more
fungal hyphae associated with them. |
Pesticide Inputs
There is no single explanation of how pesticide inputs (herbicides, insecticides and fungicides) affect soil biology and there is very little data available from an Australian agricultural context. Pesticide effects could be either reversible or irreversible. In most cases the effect of a single application of a chemical is reversible. Effects may be irreversible if the mode of action of a chemical also applies to a microbial biochemical process. For reversible effects it is important to know the magnitude and duration of the effect in order to manage the effects relative to biological recovery. The impact of a pesticide on beneficial soil biota depends upon the rate of degradation which is influenced by overall level of soil microbial activity. Generally it is considered that there are few long term effects caused by herbicides, whereas negative effects of insecticides and fungicides on non-target organisms are more common. Copper based fungicides are among the most toxic and persistent chemical inputs.
Herbicide use is a necessary management practice in modern cropping systems such as no-till systems. When more than one herbicide is used in a crop season, it is important to choose the herbicides applied in succession to avoid long periods of negative effects on microbial activity.
Agricultural chemicals are broken down in a similar way to organic matter where microorganisms produce the appropriate enzymes to degrade the compound. More complex structures degrade more slowly either because fewer microorganisms in the soil produce enzymes capable of degrading them or because of the inaccessibility to microbes. When agricultural chemicals are degraded, the microorganisms responsible obtain carbon (energy) from the chemical allowing these microorganisms to grow and multiply. Microorganisms capable of degrading a particular chemical grow and multiply until there is a higher proportion of them in the soil relative to other species. This can result in this community of microorganisms in the soil adapting to the point where the chemical can be broken down more rapidly than it would have previously. This can be a problem when rapid degradation of a chemical may reduce its effectiveness against pests.
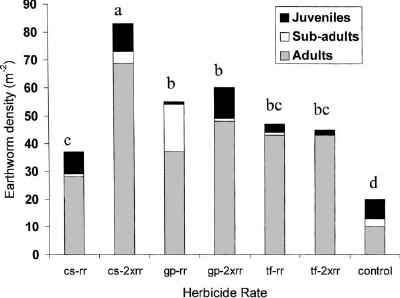
P.M. Mele, M.R. Carter ; Soil & Tillage Research 50 (1999) 1-10 |
- Earthworm density & age structure changes with herbicide type & application after two cropping years in north-eastern Victoria. Shows that in general earthworm numbers increased after herbicide application.
- Chlorsulfuron (cs); glyphosate (gp); & trifluralin (tf) atrecommended (-rr) and double recommended (-2rr) rates of application
|
Lime
Another soil application routinely used in Australian farming systems where acidification has become a problem is Lime. Lime is applied to over 6 million hectares of agricultural land in SE Australia. The recommended rate of application is approximately 2 t/ha; this raises pH by approximately 0.5 to 1 pH unit. Lime application and the associated increase in soil pH is strongly correlated with shifts in soil microbial populations. Specifically higher activity of nitrogen fixing and nitrogen mineralising bacteria is observed with lime applications.
Sodicity and salinity
There is virtually no data available in Australia regarding the impact of sodicity and salinity on soil biology. One investigation (Wong et al., 2004) considered the effects of sodicity and salinity on soil microbial biomass. Interestingly the authors observed increases in microbial biomass with increasing sodicity and salinity. The authors suggested the large increase of microbial biomass in the high-salinity high-sodicity treatment may be due increased dissolved organic carbon from dissolving organic matter, initially providing substrate for the microbial population. In conjunction with increased organic matter solubility, high salt solutions, particularly those high in sodium can flood exchange sites on clays, causing organic carbon sorbed on to clay surfaces to be desorbed, also providing additional substrate for the microbial population. Increasing sodicity alters soil physical properties by increasing dispersion and slaking, thus causing microaggregates to disperse. Aggregates have been shown to contain organic matter in their cores, which is physically protected from decomposition. When conditions occur, which cause aggregates to disperse, the organic material in the cores becomes available for decomposition, which can be reflected in the microbial biomass.
Increasing salinity and sodicity appears to provide additional substrate for the microbial population in the soil, with the effects due to sodicity more evident than those due to salinity. This has implications for natural resource management and carbon accounting. Where salinisation and sodication of soils is occurring, soil carbon stores are most likely becoming depleted as organic matter becomes solubilised, providing additional substrate for the microbial population, while plant inputs decrease due to stresses caused by increasing salt and sodium content of the soil. Additional research is required in this area where field trials are necessary to ascertain the impacts over longer time scales due to salinisation and sodicity.
Related Links
Further information about Soil Biology.