What Regulates Soil Biology? | Measuring Soil Biology | Management and Soil Biology | Soil Evaluation of Biological Productivity
There are three components of soil biology for land managers to consider; i) the natural processes themselves (soil engineering, litter transformation and micro-food web participation), ii) the impact of management on these processes, and iii) the combined effect of the first two on plant performance). The processes that are mediated by soil biology impact on plant (and animal) production systems by modifying the soil physical, chemical and biological environment within which plants grow and persist.
Decomposing plant residues
The whole process is very dynamic – while some of the residues are being eaten and fragmented for the first time by the litter transformers, other residues have already been sequestered by soil microflora, to be in turn eaten by microfauna predators.
The process of converting plant residues (and other organic residues/wastes) into humus is facilitated by enzymes, either contained within the soil organisms or secreted by either living or dead soil organisms into the soil matrix. Some of the most common enzymes found in soils include cellulase (converts cellulose to glucose subunits), protease (converts protein to amino acids), lignase (breaks down lignin chains), urease (converts urea to ammonia and carbon dioxide) and glucosidase (converts starch to glucose). Eventually, the residues will have been processed to the point that they are relatively stable as humic substances (humic and fulvic acids). Humus represents the recalcitrant compounds of the original residues as well as the faeces and dead bodies of the soil organisms. | 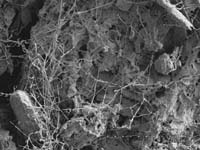
Fungal hyphae enmeshing organic residue which has been degraded by a suite of enzymes releasing nutrients into the soil matrix |
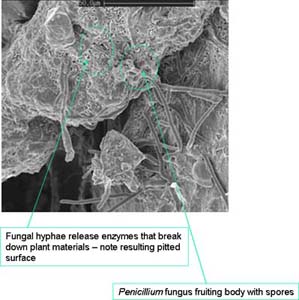 |
Fungal hyphae grow on plant residues - secreting a range of enzymes (such as cellulases, lignases and proteases) that break down these residues and release plant nutrients and gases (note pitted holes in substrate). Bacterial colonies remain attached to plant and soil surfaces and also secrete enzymes to release plant nutrients. The nutrients produced can be re-used by the microbes and are then respired or transformed into humus, taken up by living plants or lost through volatilisation or leaching. |
Carbon cycle
Soil biota play a key role in the carbon cycle whereby these organisms assimilate carbon (mainly from plant residues) and act as a large carbon sink as well as providing a substrate carbon source upon their death for other soil biota higher in the food web. The ability of soil microbes to tie up large quantities of carbon in these ‘sinks’ is becoming increasingly important in a world where greenhouse gas emissions are at the forefront of attitudes and policies alike.
Regulates nutrient supply
Nitrogen (N) cycle
Soil biology mediates many different transformations of nitrogen in the entire N cycle. N-mineralisation and nitrification provide a useable inorganic N source for plant uptake while N fixing bacteria convert gaseous nitrogen (N2) to a useable form. These processes are fundamental to the cycle and are of obvious importance in an agroecosystem context. The ability of soil denitrifiers to release nitrogen oxides from the soil also makes these microbes important in the context of greenhouse gas emissions. Nitrification is the biological oxidation (of ammonia) with oxygen into nitrite followed by the oxidation of these nitrites into nitrates that can be used by plants. The oxidation of ammonia into nitrite is performed by two groups of organisms, ammonia oxidizing bacteria and ammonia oxidizing archaea. Ammonia oxidizing bacteria can be found among the
- and
-proteobacteria. In soils the most studied ammonia oxidizing bacteria belong to the genera Nitrosomonas. The second step (oxidation of nitrite into nitrate) is mainly carried out by bacteria of the genus Nitrobacter.
Nitrogen fixation
Nitrogen fixation is the conversion of atmospheric nitrogen (N2) into ammonia (NH3). A number of soil bacteria contain the enzyme nitrogenase that allows them to fix N2, either when free-living (Azospirillum and Azobacter) in the soil or in association with leguminous plants (Rhizobia and Bradyrhizobia). In agriculture, the association of Rhizobia and crop and pasture legumes plays a key role in land productivity. The Rhizobia colonise the legume roots and live in nodules that are formed on the roots. The Rhizobia are provided with habitat and supplied with nutrients and energy in the form of carbon compounds by the legume. In return, they fix atmospheric N2 into ammonia, which is then converted to amino acids and amides within the nodules before being transported in the xylem sap to other plant parts. The amino acids and amides are then used by the legume for growth. This mutually beneficial arrangement is called symbiosis. The fixed N used for legume growth results in reducing the need to apply N fertiliser, and the N-rich residues that remain after the legume has been harvested or grazed add N to the soil. The actual amount of N fixed is determined by how well the legume crop or pasture grows, reflecting the effectiveness of nodulation, seasonal conditions and management, and the level of nitrate in the soil at planting. Soil nitrate suppresses nodulation and N2 fixation. Thus, high soil nitrate means low N2 fixation. Subsequently, in an effort to utilise this potential, screening for ‘elite’ Rhizobia is occurring in order to isolate effective N-fixing strains for newly released legume cultivars (including natives) as well as efficacious N-fixing strains for stressed soil conditions & dry-sowing (drought tolerant). Research is also being undertaken to optimise ‘delivery’ systems where carrier materials and preparations such as peat, granules and polymers as well as furrow injection technologies are being investigated.
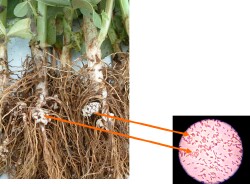
This figure shows the “pink” nodules on legume roots where N-fixing bacteria live in a symbiotic relationship with the host plant. The “pink” colour indicates the bacteria are actively fixing N. “White” coloured nodules might indicate the bacteria are not fixing any N. | 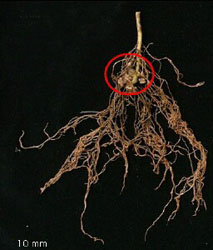
Crown nodulation, typical of peat inoculants but less
efficient at N-fixation | 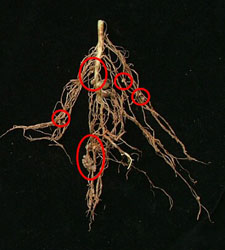
Crown and deep nodules from effective granular and
liquid inoculants |
This figure highlights the enzymatic potential of many soil microbes to access “locked-up” P in the soil matrix therefore making the P available for plants to utilise.
| Phosphorus supply
Soil biology is crucial in the functioning of the phosphorus cycle. Different organisms are responsible for the mineralisation of organic phosphorus from organic to “useable” inorganic forms suitable for plant uptake. 70 - 80% of identifiable free living soil microbes, particularly bacteria and fungi, produce enzymes involved in phosphorus mineralisation (e.g. phosphatase, phytase, etc). Large quantities of phosphorus are also immobilised by soil microbes as it is a major component of two of the most important molecules in all living things: ATP (natures ‘fuel’) and DNA (the building block of life). |
Mycorrhizas
Mycorrhizas are the associations plant roots form with fungi and are more often than not beneficial for both partners. The most common type of mycorrhizas are the arbuscular mycorrhizas (AM) involving members of the phylum Glomeromycota. Many plant species from both natural and agricultural ecosystems form these mutually beneficial symbiotic associations.
The AM fungi form a hyphal link between the host root and the soil. The fungi receive carbon sources as energy from the host plant and in return the extensive fungal hyphal network assimilates and transports nutrients particularly phosphorus and to a lesser extent micronutrients such as copper and zinc back into the plant roots from the soil. Arbuscules (which give the mycorrhizas their name) are “tree-like” branched hyphal structures formed in the host root cells and are considered the major site where carbon and nutrient exchange between plant and fungus occurs. AM increase the volume of soil explored by the plant well beyond the root zone, a trait that is especially relevant in Australian soils which are characteristically low in phosphorous. It is widely accepted that AM also increases water use potential by the host plant, improve the hosts ability to deal with salinity and help suppress some common pathogens.
Conventional agriculture practices, such as tillage, heavy fertilizer and fungicide use and poor crop rotations hinder the ability of plants to form symbiotic associations with AM fungi. There is great potential for low input agriculture to manage the system in a way that promotes mycorrhizal symbiosis, although all agroecosystems can benefit by promoting AM establishment. Proper management of AMF in the agroecosystems can improve the quality of the soil and the productivity of the land. Agricultural practices such as reduced tillage, low phosphorus fertiliser usage and rotations promote beneficial mycorrhizal symbiosis.
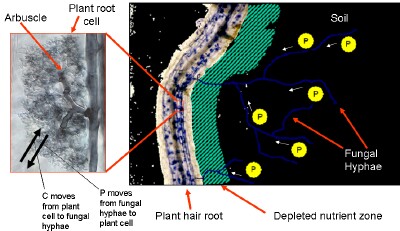
This figure shows how arbuscular mycorrhizal fungi form a symbiotic association with many crop species.
The fungi increase the plant hosts access to P and in return obtain a C source from the plant.
Improved soil structure
Soil biota can have a direct effect on soil structure. Soil aggregates are clusters of soil particles comprising sand, silt, clay particles, humus and soil organisms. Aggregation of the soil is important for maintaining porosity and for stability of surface soils against erosion. The stability of aggregates is related to the level of soil organic matter and to the activity of soil organisms. For example, filamentous fungi, particularly mycorrhizal fungi, bind particles of soil into stable aggregates through physical entanglement. Bacteria also help stabilise smaller aggregates (2-250 µm) due to attractive forces of chemical charges on their surface. These smaller aggregates may subsequently be bound together into larger aggregates (>250 µm) through the action of fungal hyphae. Soil biota bi-products can also have a direct effect on soil structure. For example, glomalin is a glyco-protein produced by the common root-dwelling AM fungi, Glomerales, binds soil particles and organic matter to form soil aggregates – this compound is referred to as the “soils superglue”. Polysaccharide exudates from bacteria also act as “super-glue” holding soil particles together to form aggregates.
 | 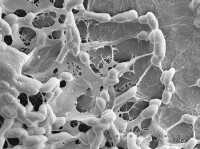
Bacterial “glue” composed of polysaccharide exudates | 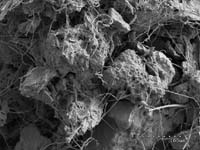
Fungal hyphae enmeshing soil aggregates. This helps form pores (macro and micro) that increase aeration and water infiltration in soil |
Degrades chemicals inputs
Agricultural pesticides (herbicides, insecticides and fungicides) are degraded in the soil principally by the action of microorganisms, a process termed biodegradation, where biodegradation is defined as the breakdown of a substance to smaller products caused by microorganisms or their enzymes. The rate at which different pesticides are biodegraded varies widely but generally degradation is faster in soils with high microbial diversity and activity. Some pesticides such as DDT have proven to be recalcitrant. Consequently they remain in the environment for a very long time and are known to accumulate into food chains decades after their application to soil. Pesticides which are more readily biodegradable, such as the organophosphates, are now used in preference to the more persistent chlorinated pesticides. Others, such as atrazine and simazine, are biodegradable at slow rates and may be leached from soil to ground water, where they pose a threat to drinking water supplies. At the other end of the scale, there are pesticides, such as carbofuran and diazinon that are readily biodegraded, and are broken down so rapidly under certain soil conditions that they may not allow effective pest control. Furthermore, toxic elements, e.g. arsenic, chromium and mercury, can be ‘locked’ in the soil by microbial activity, preventing further pollution.
Biocontrol
Many soils support microbes that are deleterious to plant growth; the symptoms can be visible (clinical) or invisible (sub-clinical). Soil fumigation has quantified the link between soil microbes with crop yield performance. A variable impact on grain yields has been demonstrated with up to an 80% increase in yield on highly alkaline Calcarosols (D. Roget) & up to 60% reduction in yields on highly acidic Dermosols (P. Mele). Why the variability in response? – fumigation could actually knock out beneficial organisms in a disease suppressive soil allowing a disease causing agent to proliferate in the soil. Organisms identified as potential biocontrol agents in suppressive soils include the soil fungi, Trichoderma spp, Penicillium spp and Metarhizium spp and the bacteria, Pantoea, Exiguobacterium and Microbacterium. Reported modes of action of the suppressive microorganisms are production of antibiotics, competition for infection sites on the roots, competition for space and nutrients in the rhizosphere and parasitism of the pathogens. These beneficial organisms should be encouraged through management such as crop rotations and maximizing the return of residues (carbon source) to the soil in order to restore a balance between the soil biota in the soil so that pathogens do not dominate. Disease suppressive soils are more frequently being identified and soil-borne pathogens such as Rhizoctonia, GG Take-all & Pythium can be controlled in these soils. A suppressive soil is one that, despite having conditions that favour the establishment of a disease-forming organism, results in the pathogen:
- either not establishing,
- establishing, but not producing disease symptoms,
- establishing and then declining.
A suppressive soil does not result in the elimination, but the control of disease forming organisms. Farmers should be trying to achieve a level of suppression (microbial balancing) that prevents disease. But suppression is relative - a soil may be suppressive, but disease at a lower level is still experienced.
Greenhouse gases
Primary industries are significant emitters of greenhouse gases. Agriculture is the third largest contributor to Victoria's total greenhouse gas emissions. Therefore, this sector has an important role to play in reducing Victoria’s emissions.
Nitrous oxide emissions are produced by a range of idenitrifying bacteria in the soil, where they convert nitrate into nitrous oxide. These losses are greatest when soils are warm and waterlogged, and in those with high nitrate contents. Thus it is important to apply nitrogen fertilisers only at times, and in quantities and forms, useful to plants as overuse of fertiliser can drastically increase levels of denitrification. Nitrous oxide is a more potent greenhouse gas than carbon dioxide. One unit of nitrous oxide is equivalent to 310 units of CO2. Conventional tillage systems also release more CO2 into the atmosphere than no-till systems as the incorporated residues turnover more quickly and result in more carbon being respired by the microbial community. No–till systems tend to lock up more carbon in the form of organic matter. A large soil microbial community can also tie up carbon and nitrogen that might otherwise be released into the atmosphere as greenhouse gases.
Related Links
Further information about Soil Biology.